By Ken Jakalski, Head Track Coach, Lisle High School.
Don’t Hit Like Da’ Preem
At his recent Speed Summit presentations in Lisle, Illinois, SMU’s Dr. Peter Weyand discussed the implications of the SMU lab group’s latest papers, “Foot speed, foot-strike and footwear: linking gait mechanics and running ground reaction forces” and “Are running speeds maximized with simple-spring stance mechanics?” In the second paper, Ken Clark, Laurence Ryan and, Weyand hypothesized that a passive, linear-spring model, long regarded as the means by which runners optimize force production and economy, would not apply to the mechanics that maximize the ground forces essential to high speed running.
That alone posed a research challenge, since this classic model seemed to make so much sense. Early in the stance phase, the runner’s leg is compressed as a result of the downward force of gravity. This downward force stores energy in elastic tissues of the limb and then, during the latter portion of the stance phase, this energy is released through elastic recoil. The model is both simple and elegant.
But apparently, it’s not the model that applies during high-speed running.
As the authors note, “The across-speed results obtained from the competitive sprinters suggest that deviating from a simple-spring pattern of ground force application may be a mechanism these athletes used to attain faster speeds.”
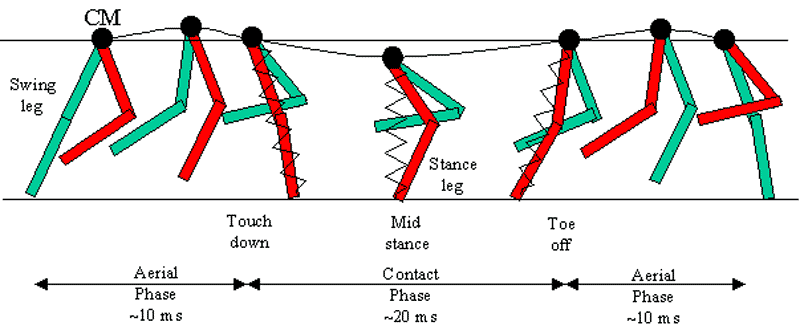
Figure 1: Gait Cycle
So if it don’t mean a thing if you ain’t got the spring—at least relative to high speed running—what IS going on?
The authors noted that waveforms during high speed sprinting are asymmetrical, which means they are not fully consistent with the patterns of the long held spring-mass model. But what do these waveforms of the fastest look like? Quite simply, the researchers observed a much steeper rising edge and peak force occurring well before mid-stance with this force greater in magnitude at faster speeds. How much earlier in the stance phase are these forces peaking? The research reveals force peaks occurring at 25% of contact time, with some sprinters exhibiting two force peaks, with the first occurring in the first 20% and the second around 50% of contact time. The take-home message is clear. For elite sprinters, the faster they run, the less their waveforms resemble those of the traditional spring-mass model.
And now, the million dollar question which will be posed to the researchers in the months and possibly years ahead: What are the implications of this research relative to the way we train athletes to achieve faster top end speeds? In other words, how can our sprinters duplicate that asymmetric waveform that looks considerably different from the simple-spring pattern we see at much slower speeds?
Dr. Weyand did address that question at the Speed Summit. Two things appear to have caught the attention of the researchers. First, high knee lift in the late swing phase allows for greater limb velocity prior to landing. Second, the erect posture we see in the fastest sprinters appears to contribute to the stiffness required to decelerate the limb and body right after ground contact.
And from these observations, the take-home mechanics message is powerful in its simplicity: As Weyand noted, “These big forces are largely accomplished in the wind up and delivery of force to the ground in the impact phase – like throwing a punch with the foot to the ground.”
So, coaches should picture the limb literally delivering a punch to the ground…but not just any punch.
What should that punch look like?
To answer that question, I’ll offer the following analogy to the famous Ambling Alp of the boxing world, Primo Carnera. Da Preem was a gigantic man mountain (he actually suffered from both pituitary gigantism and acromegaly) He certainly had great mass and unusually long levers, but he was NOT a devastating puncher.
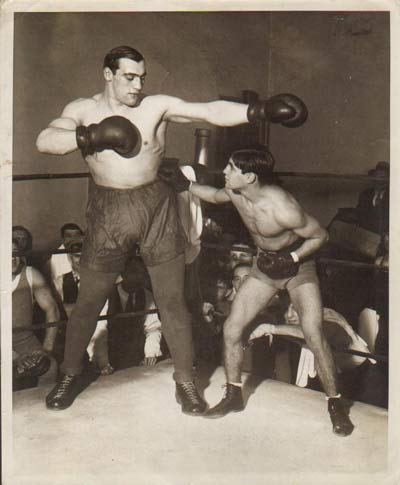
Figure 2: Carnera and Singer
I think the parallel to sprinting is that the lack of force in Primo’s punches was tied to the relationship between mass and acceleration. A forceful punch in boxing depends not on pure strength but in learning to get the body behind the punch and to accelerate the arm to the target. Primo certainly had mass (265 pounds) and long levers (an arm span of seven feet), but his punches came in slow and were easy to avoid.
And not only were his punches slow enough to be avoided, but they were not as devastating as many would believe. Size does not equal punching power. Also, strength does not equal punching power. If either were true, Primo Carnera would be one of the hardest punchers of all time.
And that’s because slower punches lack force. Boxing experts compared Primo’s punches to a wrecking ball. Once set in motion, a wrecking ball could plow through concrete and steel. But Primo’s punches lacked mechanical advantage. It was not his fault he would never become a great puncher. The laws of physics and the “geometry of his prodigious size” worked against him. He couldn’t overcome the enormous inertia of his oversized body.
As neurologist Harold Klawans notes, “slowing the punch down to one-third of the speed at which it should be traveling markedly decreased the likelihood of da’ Preem ever delivering a haymaker.”
Sprinters, in terms of force on the ground, need to deliver a specific kind of haymaker. How the leg gets to the ground in sprinting is very much like how the fist of a boxer gets to the opponent’s face.
To achieve this, the sprinters need to consider how, where, and when they are foot-punching the track. This involves a focus on three things: posture, front side mechanics (Ralph Mann’s approach going back to the 80’s) and foot-strike. Researcher Ken Clark points to the value of focusing on aggressive front-side mechanics. “This,” he notes, “has a two-fold beneficial effect. Powerful hip flexion and high knee lift of the front thigh, and simultaneous powerful hip extension of the rear thigh results in large forces and short contact times.”
So, for sprinter to deliver a knockout time, it’s not in the mass and levers of a Primo Carnera, but in the position, coordination, and timing of a Bruce Lee.”
https://www.youtube.com/watch?v=_BRB3FJ8IVs
Please share so others may benefit from this article.
[mashshare]References:
Clark, Kenneth P., and Peter G. Weyand. “Are running speeds maximized with simple-spring stance mechanics?.” Journal of applied physiology (Bethesda, Md.: 1985) (2014).
Clark, Kenneth P., Laurence J. Ryan, and Peter G. Weyand. “Foot speed, foot-strike and footwear: linking gait mechanics and running ground reaction forces.” The Journal of experimental biology (2014): jeb-099523.
Klawans, Harold L. Why Michael Couldn’t Hit: And Other Tales of the Neurology of Sports. New York, NY: W.H. Freeman, 1996. Print.
Hello Ken:
According to my research (and the reading of recent specialized articles), maximizing running speed is more related to being able to _keep_ relatively large horizontal forces (and power) at high speeds. The previous idea is predicted by the spring mass model. Humans seem to be somewhat deficient at developing large horizontal forces at higher speeds, and that probably explains why we are considerably slower than other expert sprinter animals of roughly the same mass.
However, it seems likely that the super elite human sprinters DO optimize their horizontal propulsive force by hitting the ground during the landing phase (as Weyand 2014 has found in the article you quote), but only in order to store elastic energy in tendons, This energy storing is likely to be released during the propulsive phase of the contact time by means of improved horizontal work, horizontal force and horizontal power.
Best regards,
Mauricio A. Fuentes
It’s good to see a little more progress made to understanding what is involved in sprinting or running in general. However some of the key factors are still being overlooked.
The main reason for this I believe is the hang up on vertical force produced on contact. Noticed how the mechanism of this is still ignored as well as what happens to this force during the stance phase. Where and when will horizontal forces be taken into consideration?
Hip flexion and extension are mentioned but still ignored in regard to the role that they play. If we would look more closely at their role we would move away from our blindsided limitations to vertical force on contac.
I strongly recommend reading Explosive Running for more information on the role of hip flexion and extension and the production of horizontal force
Completely false, and a common misconception. It is not horizontal force that creates speed but vertical, many studies have shown this and it makes sense in relation to physics.
A common misconception, horizontal forces are not the cause of speed therefore are irrelevant to the subject. Physically, vertical forces are the ones that produce speed
Dear Max,
I would invite you to exercise caution. The only way to “create” horizontal speed is to have horizontal forces during the acceleration phase (Newton’s 2nd law is pretty stringent in this regard). In relation to physics, the (correct) emphasis on vertical force (Ralph Mann, Peter Weyand & Co.)nhas created a few misconceptions too. At top-end speed running, *horizontal* acceleration reaches zero when once the *horizontal* forces are at equilibrium (again, Isaac Newtons’s 2nd law of mechanics, and it applies to apples, bicycles and humans alike). The horizontal forces are 1) the braking forces created during foot strike, and 2) the aerodynamic drag 3) the propulsive horizontal force generated by the sprinter. Now, aerodynamic drag increases with the square of the relative speed between the athlete and the air and effectively sets the attainable speed by a given athlete (hence the advantage given by a tailwind). When the horizontal force component can no longer exceed the sum of braking forces and drag, the athlete stops accelerating and reaches maximum velocity. At this point that vertical forces dominate over the horizontal (approx. 10:1 ratio) but the horizontal force is *far* from irrelevant. It is mechanically correct to state that, *all other things being equal*, a higher horizontal force would result in a higher top-end speed. The trouble is that all other things are not equal, because the athlete has a finite amount of force that gets “punched” into the ground, and the angle of application of the force vector determines the partition of said force between a horizontal and a vertical component. The required vertical component, in turn, is constrained by the requirement to reverse the vertical velocity of the center of gravity during the contact phase (since the CoG “rises and falls” by about 10cm after each foot contact phase, during the flight phase).
The available horizontal force is “what is left” once the above vertical requirement is met.
So strictly speaking, vertical an horizontal force are not independent of each other. If the angle of force application is the same, it is mechanically impossible to increase vertical force without “surreptitiously” increasing the horizontal force available to the athlete (which needs to be greater then zero, to compensate for the loss of momentum caused by braking forces and drag).
The matter is further complicated by the interplay between vertical force (and stride rate) and horizontal force (and stride length). While the general principles are clear (such as the importance of vertical force in attaining short contact times and higher stride rates), it is also clear that not all athletes “solve” the problem exactly in the same way (compare Leroy Burrell’s 9.85 run to Justin Gatlin’s 9.85 in Athens, and you will see they were pretty different sprinting animals, in spite of their similar body types and of clocking identical times).
In short: I personally feel that “vertical force” is dangerously close to becoming a slogan, while the matter deserves to be treated with some added context. And some fine points are far from exhaustively resolved.
Kind regards,
Max